Projects
Thermodynamics and transport
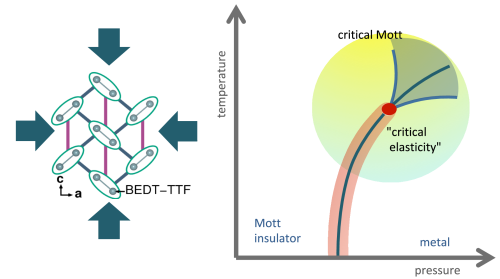
In this area, the focus lies on materials design and synthesis, thermodynamic characterization and analysis of transport properties, in many cases under applied stress. Of crucial importance will be to gain control over the various phases by using suitable tuning parameters that couple to the materials' elastic strain. Depending on the material and the purpose, this can be chemically induced pressure (mainly via isoelectronic substitutions), hydrostatic pressure, uniaxial- or biaxial strain, or a combination thereof. In this way, we will be able to disentangle effects of competing interactions and to study their role for the material's property of interest.
Strong electron-lattice coupling in correlated intermetallic compounds near valence- and structural instabilities
A01 (Wolf/Lang) will study the close interlocking of electronic and lattice degrees of freedom upon pressure-tuning systems into the vicinity of phase transitions. Special attention will be devoted to the expected occurrence of critical elasticity around the valence transition in EuPd2Si2 and related variants. A second focus will be the possibility to induce novel phases, such as valence-fluctuation-induced superconductivity, near the transition associated with the collapse of the tetragonal unit cell in Eu(Fe1-xNix)2P2.
This project ends with the first funding period on June 28th 2024.
Uniaxial- and biaxial-strain-induced phase tuning of correlated metals
The project will tune layered Kagome metals AV3Sb5 (A = K, Rb, Cs) and the AEMn2P2 (AE = Sr, Ca) magnetic intermetallics, i.e. systems with complex electronic orders using a combination of strain, doping and/or hydrostatic pressure to understand the role played by the structural and electronic degrees of freedom in the stabilization of complex emergent phases.
Single crystal growth of correlated intermetallic compounds with strong electron-lattice coupling
The project will grow and characterize intermetallic compounds to study collective phenomena where both lattice and electronic degrees of freedom are involved. The project aims to provide single crystals of highest possible quality. In addition, an explorative growth of new correlated flat-band materials in systems with two-dimensional Kagome structural motives will be performed as well as a search for intermetallic materials showing altermagnetic order.
Strain effects in thin films of correlated intermetallic compounds
The project will concentrate on materials with pronounced spin-lattice coupling and intertwined crystal symmetry and magnetic order in the form of epitaxial thin films grown by molecular beam epitaxy. In particular, we will focus on the non-collinear ferromagnet LaMn2Ge2 that shows a large topological Hall effect in single crystals, the new intermetallic compound EuPdSi3 that lacks a mirror plane in comparison to the valence-crossover compound EuPd2Si2 and features two antiferromagnetic phase transitions, and Mn5Si3 with the aim to engineer a strain-induced altermagnetic state by a novel Si-membrane based pressure tuning approach. Coupling of dipolar magnons to surface acoustic waves and non-reciprocity effects will be studied in the La-Mn-Ge thin films.
Interplay of lattice, charge and spin degrees of freedom from first principles
The project will explore and model, under various conditions of anisotropic strain, pressure and doping, the role of electron-lattice coupling versus electron-electron interactions in (i) the formation of charge density wave (CDW) instabilities and superconductivity in layered Kagome-based systems, (ii) the formation of multiferroic phases in triangular-lattice organic charge-transfer salts, (iii) the competition of magnetic, structural, nematic and superconducting phases in SrRu2O4 and AT2X2 (A = rare-earth or alkaline-earth metal, T = transition metal, X = P, As) with a special focus on the phenomenon of superelasticity in some families of the latter. For that we will employ a combination of ab initio-based methods with recently developed many-body techniques.
Elastic effects in strongly correlated molecule-based systems with geometrical frustration
A06 (Lang) will investigate the intimate coupling between the electronic and elastic degrees of freedom in frustrated organic charge-transfer salts via thermal expansion and dielectric measurements controlled by temperature and He-gas pressure. Special attention will be dedicated to the phenomenon of critical elasticity around the Mott transition, and the possibility to realize and control new phases such as electronic multiferroicity and charge-glass states. There will be strong interactions with various experimental and theory projects working on these systems. The project will also supply single crystals to the collaborating projects.
This project ends with the first funding period on June 28th 2024.
Theoretical approaches to electron-phonon coupling in strongly correlated systems
The project will develop and exploit theoretical methods, such as functional renormalization group and strong-coupling expansions, with the particular focus on advancing the many-body description of altermagnetic systems. The project combines microscopic approaches and phenomenological theories of the formation and elastic coupling of altermagnetic d- and f-wave magnetism.
NV-center spectroscopy for strain sensing of non-collinear antiferromagnets
A08 (Sürgers/Wernsdorfer) will exploit the unique sensitivity of nitrogen-vacancy centers in diamond to magnetic fields, strains, and temperature to optically detect local strain fields. This new development of the method will be applied to antiferromagnetic thin films with a non-collinear magnetic structure and strong magnetoelastic coupling. In the long term, the technique will be available to other projects of the CRC/TRR.
This project ends with the first funding period on June 28th 2024.
Anomalous magnetoelasticity in altermagnets and antiferromagnets
The project will investigate primarily the recently discovered altermagnetic class of materials with compensated magnetic order, focusing on the strong coupling between crystal structure and magnetism. The project will identify unique magnetic–shear strain cross-effects characteristic of altermagnets and their unconventional magnetic order. We will search for altermagnetic material candidates, identify their magnetic ground state properties, and calculate the electronic structure coupling to strain from first principles, such as pressure-induced altermagnetism. The unconventional piezomagnetic and anomalous Hall response of these selected altermagnets with strong magnetoelastic coupling will be also calculated.
Stress-strain measurements and development of a thermodynamic elastoscope
The project will use high-precision bulk thermodynamic probes and symmetry-sensitive optics combined with uniaxial pressure to characterize and control ordered states in systems with strong coupling between electronic, magnetic, and lattice degrees of freedom. We aim to develop and make a first demonstration of ‘elastoscope’ combining spatially resolved optics, thermodynamic measurements, and in-situ uniaxial pressure.
Quantum materials with strong elastic coupling
The project focuses on quantum materials with a non-perturbative elastic coupling. Considering materials close to a quantum-critical point, the project will theoretically investigate the coupling of critical fluctuations to crystal elasticity. Two examples will be considered, the isotropic ferroelectric quantum critical point and the Lifshitz transition of a two-dimensional metal, with a particular focus on the stability of the crystal lattice in the presence of these critical fluctuations.
Strain control of altermagnetic devices
The joint theory-experiment project will explore how selected strain affects the key altermagnetic properties in a choice of materials and measure the changes to the electronic and magnonic-band splitting. We will use direct spectroscopy and transport measurements, complemented by theoretical modeling. The project will explore how strain can be used to trigger phase transitions between altermagnetic and other phases, and will study a piezomagnetic effect that is unique to altermagnets.
Elastically-tunable electronic orders in correlated electron systems under a.c. uniaxial pressures
The project will utilize the novel capability to apply a.c. strains and stresses to experimentally determine various thermodynamic response functions of selected correlated electron systems under controlled uniaxial pressure. The project aims to accomplish the following scientific goals: (i) precise control of the triangular-lattice geometry of organic charge-transfer salts to study the interplay of charge, magnetic and lattice degrees of freedom under varying degrees of geometrical frustration; and (ii) search for the proposed phenomenon of viscoelasticity, i.e., low-frequency lattice dynamics, in correlated materials with critical elasticity.
Dynamics
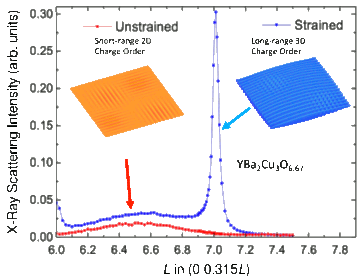
In this area, we focus on spectroscopic studies of strain-tuned materials and on the dynamic interplay between the elastic deformations of the lattice and the coupled electronic orders. Our aim is to determine pathways for dynamically controlling the electronic properties by using selective elastic stimuli.
Dynamics and noise of disordered strain-coupled electronic order
The project will develop the foundations for the quasi-classical kinetic theory of the low-frequency elastocaloric effect and for the dynamics of visco-elastic stress correlations and noise in electronic quantum phases of matter. Additionally, we aim at a quantum kinetic approach beyond the Luttinger paradigm to describe dynamic stress correlations will be. Finally, we aim to develop and analyze nonlinear two-dimensional spectroscopy as a tool to probe the dynamics of strongly coupled electron-lattice systems.
Interplay of slow charge carrier dynamics and elastic effects in correlated multi-phase systems via noise spectroscopy
The project will apply conductance and resistance fluctuation (noise) spectroscopy, under both hydrostatic and uniaxial pressure, to investigate the slow fluctuations that arise in different phases of correlated electron systems, or drive electronic or magnetic transitions, which are coupled to the elastic degrees of freedom. Target materials are (i) organic charge-transfer salts as bulk crystals and thin film field-effect-transistor structures where peculiar electron-glass states will be explored dependent on the lattice anisotropy, electronic ferroelectricity exhibiting dipolar glassiness, and band-filling-induced Mott criticality. In (ii) antiferromagnetic EuT2X2, showing colossal magnetoresistance effects, the project will study the origin of large fluctuation effects and their anisotropy by probing the coupling to uniaxial strain aiming at enhancing the magnetic properties.
Elastic tuning of competing orders in quantum materials
The project will investigate the interplay between charge ordering and other competing electronic phases in selected families of quantum materials by probing their response to elastic tuning. To this end, the project includes the synthesis of single crystals, their structural characterization and the study of their collective excitations by means of inelastic-photon-scattering techniques under uniaxially- and hydrostatically-pressurized conditions.
Momentum microscopy of strongly correlated systems under strain
The project will develop and exploit experimental methods to improve the efficiency of spin- and angle-resolved-photoemission spectroscopy based on time-of-flight-detection schemes for the study of elastic coupling of electronic states. Emphasis will be placed on hard X-ray photoemission spectroscopy to probe bulk properties as opposed to interfacial phenomena. The project aims to study the interplay between electronic order and elastic structural properties in the two-dimensional dichalcogenide and Kagome lattices, in the three-dimensional tetragonal 122 systems, and in altermagnetic materials.
Correlations and altermagnetism in elastic tunable electronic systems
The project will explore the effects of correlations on altermagnetic phases in selected weakly to strongly correlated materials by combining density functional theory and many-body methods such as dynamical mean field theory, two-particle self-consistent approach and variational Monte Carlo. We will also study the sensitivity of correlated altermagnets to strain fields and evaluate magneto-optical response functions, spin and orbital susceptibilities, and spectral functions and compare with experimental observations.
Static and dynamic coupling of lattice and magnetic properties in low-symmetry two-dimensional materials
The project will explore the physics of complex magnetism and its coupling to lattice degrees of freedom in layered low-symmetry two-dimensional materials. By combining the density functional theory approach with real-space scanning tunneling microscopy (spin-polarized and inelastic), the project will address the emergence of complex magnetic states, magnonic excitations, phononic properties and physics of magnon-phonon coupling and formation of chiral hybrid excitations mediated by orbital magnetism at surfaces of Kagome metals, honeycomb materials and altermagnets.
Phonon-driven control of electronic properties in hybrid perovskites and organic charge-transfer salts
B07E (Kim/Bonn) will study phonon-induced non-equilibrium phase transitions in two model systems exhibiting strong electron-phonon coupling. Using non-linear THz/vibrational spectroscopy, the project will explore metastable, switchable transient atomic arrangements as well as phases that are only present for the duration of the light pulse and/or the time scale over which coherent phonon motion is preserved. These investigations will be performed for κ-phase organic charge-transfer salts and hybrid organic-inorganic perovskites. The latter material class is specially chosen as a testbed case for such phenomena.
This project ends with the first funding period on June 28th 2024.
Ultrafast spectroscopy and manipulation of coupled electronic/lattice orders
The project will use femtosecond real-time approaches to study collective modes and their nonlinear couplings in selected systems with orders resulting from strong electron-lattice coupling. The project will focus on the evolution of couplings across the rich phase diagrams by tuning pressure(strain) and temperature, focusing on the vicinities of critical points. Near the phase boundaries between different, nearly degenerate ground states, we aim to utilize nonequilibrium pathways to generate metastable states with novel functionalities which cannot be reached through thermodynamical pathways.
Dynamics of strongly coupled electron-phonon systems
The project will develop a theoretical description for time-dependent processes in strongly-coupled electron-phonon systems. A specific focus will lie on the interplay of different ordered states, like CDW states or superconductors. Based on preliminary work in this area, the project will investigate the dynamics of collective modes after a pump or in the presence of a drive. Moreover, we will address how polaronic dressing can influence driven electronic states. The project aims to improve the understanding of experimental investigations, such as ultrafast optical and THz pump-probe spectroscopy as well as 2D spectroscopy.
Tuning dimensionality in low-dimensional systems by strain
The project will develop a novel methodology to apply strong out-of-plane strain and strain gradients to structurally layered single crystals. This will drive systems closer to a 2D limit, which impacts and usually enhances correlation effects. Fe-based, nickelate and ruthenate unconventional superconductors will be targeted, systems in which low dimensionality is believed to be key to their superconductivity. Such experiments are strongly hindered by the tendency of layered materials to cleave under tension, an experimental obstacle we aim to overcome by developing a novel approach based on microfabricating crystalline pillars with a strong amorphous coating to prevent fractures from propagating. We will probe the response of correlated phases using primarily transport and Raman spectroscopy.